After the discovery of the high-temperature superconductors, their parent compounds are conjectured to be in a spin liquid phase which becomes superconducting when charge carriers are doped. Spin liquid is a highly entangled quantum disordered state. Due to its strong geometrical frustration, the Kagome quantum magnets are expected to host such novel phase. Searching for an ideal Kagome quantum magnet has led to recent discovery of new materials from structural polymorphs of herbertsmithite, i.e., Ca-kapellasite which is described in Fig.1(a) and (b).
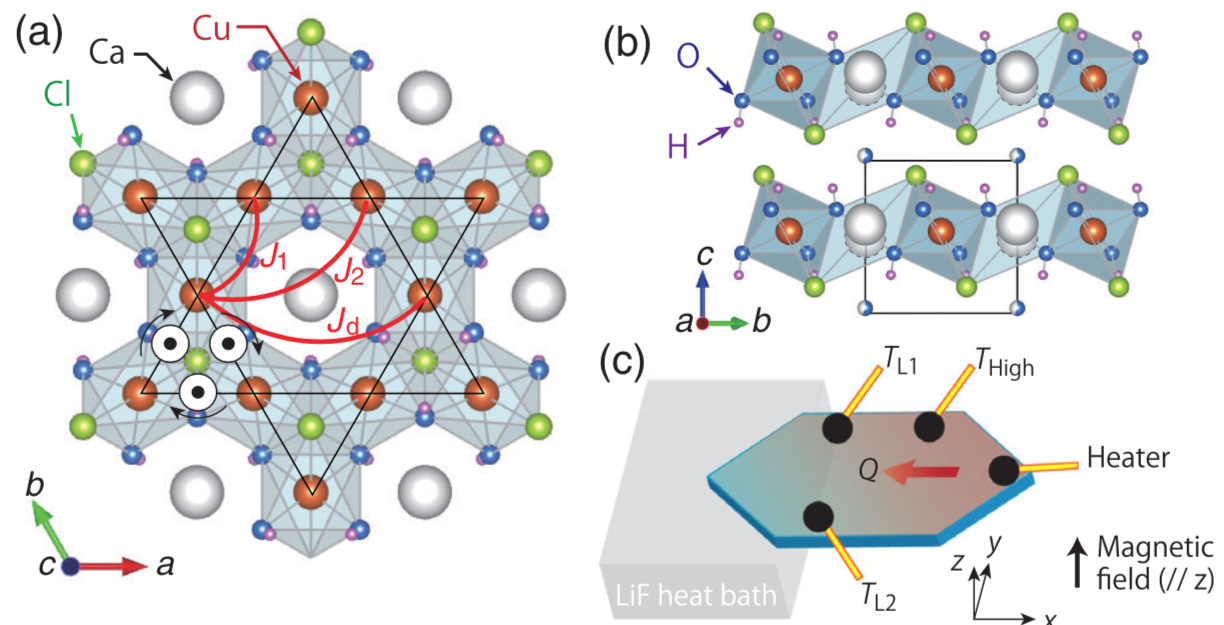
However, according to exhaustive theoretical investigations, it may host many different types of spin liquid phase including RVB, Z2 and Dirac spin liquids. Therefore, it is very important to find the elementary excitations expected to emerge in these unknown phases through experiments on ideal Kagome materials, though it has been remained as a very challenging problem. As an evidence of emergence of spin liquid phase and its excitation, the thermal Hall response, which is an analogue of Hall response in electronic system, has been suggested and, in fact, the finite thermal Hall conductivity was measured. However, its origin was not so clear since the phonon also can transport the heat.
In this study, a clear thermal Hall signal was observed in the spin-liquid phase of the S=1/2 kagome antiferromagnet Ca-kapellasite and provide a strong evidence suggesting the heat is carried by the spinon excitation on top of the spin liquid state. In order to obtain a theoretical estimation of thermal Hall conductivity, we employ the Schwinger-Boson mean field theory (SBMFT) and the U(1) spin liquid ansatz. The thermal Hall conductivity can be measured by the following formula
Where c2 is a distribution function of the Schwinger boson, and nB the Bose-Einstein distribution function, E is the Schwinger boson band, and Ω is the corresponding Berry curvature. Remarkably, the (dimensionless) thermal Hall conductivity obtained from the SBMFT is perfectly fitted by the ones obtained from the experiment as shown in Fig. 2.
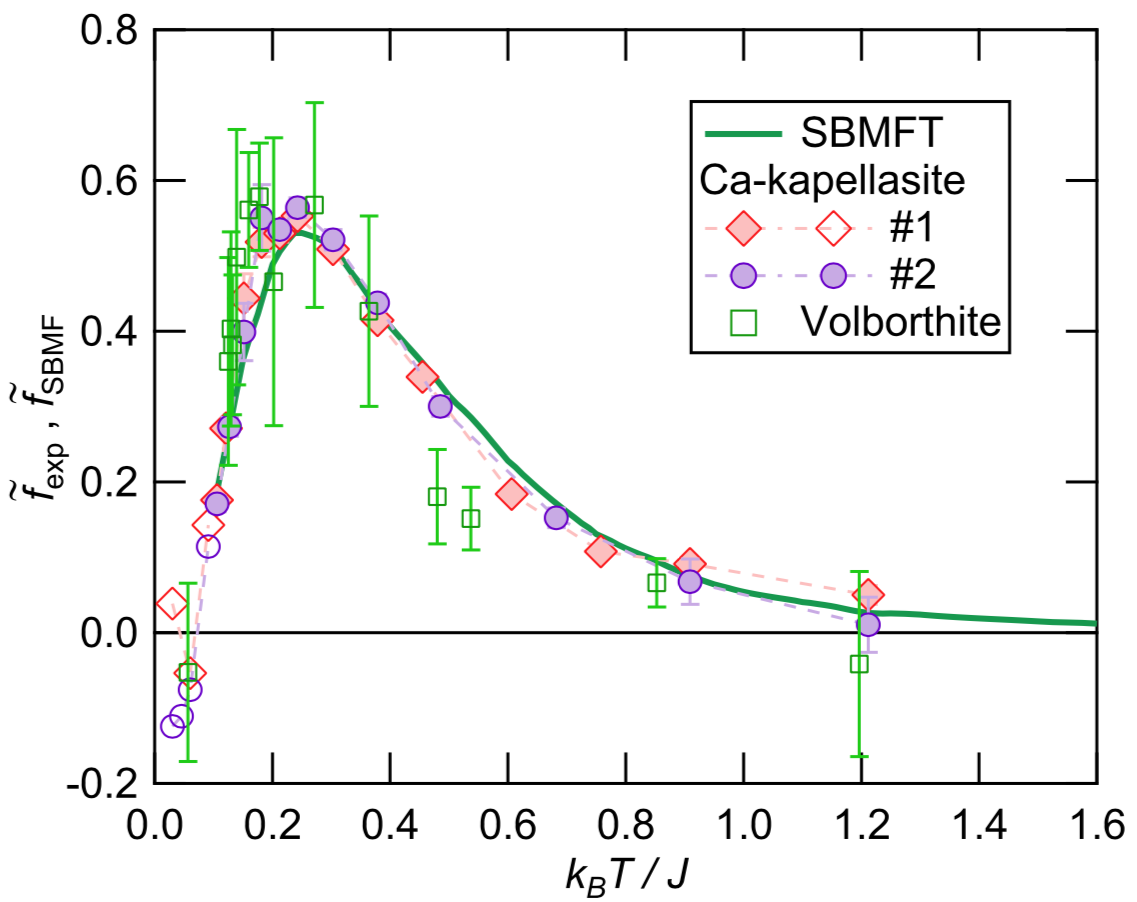
Also, we find all observed thermal Hall conductivity curves from many samples converge onto a single curve by adjusting only the exchange interaction and Dzyloshinkii-Moriya interaction, which suggests that it has a common temperature dependence on the Kagome quantum magnet. This excellent agreement, both qualitative and quantitative, demonstrates that the thermal Hall effect in these kagome antiferromagnets derives from spins in the spin-liquid phase.
Reference
Hayato Doki, Masatoshi Akazawa, Hyun-Yong Lee, Jung Hoon Han, Kaori Sugii, Masaaki Shimozawa, Naoki Kawashima, Migaku Oda, Hiroyuki Yoshida, and Minoru Yamashita, Phys. Rev. Lett. 121, 097203 (2018)